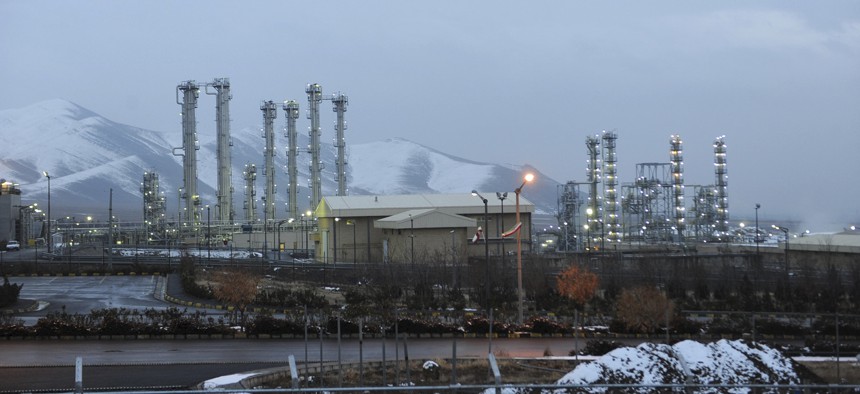
A view of Iran's heavy water nuclear facilities is seen, near the central city of Arak, Saturday, Jan. 15, 2011. Hamid Foroutan/AP
How Antimatter Could Stop Iran From Cheating On the Nuclear Deal
Advanced particle detectors could be the key to ensuring the success of the agreement.
The West’s deal with Iran is based on the premise that international monitors at the IAEA would be able to detect illicit refinement of weapons-grade material — no easy task when the landscape includes radioactive detritus from previous nuclear efforts. But technologies now in their infancy, could someday help reveal if Iran resumes its pursuit of a bomb.
Consider the IR-40 heavy water reactor in Arak, which can produce some 10 kilograms of weapons-grade plutonium a year. Under the deal, the reactor would be altered to perform engineering and research work, but the radioactive traces of its original purpose might easily confuse today’s sensors, leading to uncertainty about Iran’s ongoing efforts. “They enriched uranium to a high level. Well, environmental sampling from now for eons will detect that.” UN weapons inspector David Kay told Defense One last week.
But what regular radiation detectors miss, antineutrino detectors could catch. Neutrinos—and their antimatter corollary, antineutrinos—are subatomic particles with no positive or negative charge. They pass through virtually all material you can imagine, from lead shielding to the cores of stars. That could allow monitors to “‘see’ into the core of a running nuclear reactor and to measure the plutonium content in situ,” said Patrick Huber, an associate professor of physics at Virginia Tech. Nuclear reactors produce antineutrinos in unimaginable quantities — up to 100 billion billion per second.
Spotting neutrinos, which move through matter without interacting with it, is very hard. You have to track these “ghost particles” indirectly, by detecting their collisions’ effects on other charged particles, and you need a lot of sensors. Examples include Japan’s Super-Kamiokande neutrino detector, which is lined with more than 13,000 sensors for observing subatomic neutrino interactions, and the lake-sized Antares detector in the Mediterranean.
But antineutrinos have a different energy profile and much larger energy flux so they are easier to detect. When an antineutrino meets a proton, the collision produces a neutron and a positron. (Positrons are the antimatter equivalent of electrons.) As positrons move through matter, they lose energy and eventually collide with electrons.
When that happens inside of a so-called scintillator material like mineral oil or plastic, these processes combine to produce measurable light. Similar flashes occur when the neutron meets with a gadolinium or lithium atom. Both of these flashes serve as a smoking gun for antineutrinos — and possible illicit nuclear activity.
In a paper published last year with several collaborators, Huber shows that it’s technically possible to detect extremely small changes in the core of a nuclear reactor, well smaller than would be relevant to making a nuclear weapon, with as much as 90 percent certainty.
Moreover, solid-state scintillators are replacing liquid ones, making it far more practical to build a deployable detector, Huber said.
“In 2011, an issue in the understanding the relation between past data and new calculations of reactor antineutrino fluxes was pointed out by a group at [the Saclay Nuclear Research Centre in France] and independently confirmed by myself,” he told Defense One in an email. “One possible and spectacular explanation would be the existence of a 4th type of neutrino, which would be an entirely new fundamental particle. Whoever can prove its existence is in line for a Nobel prize. Testing this hypothesis requires neutrino detectors which have many—if not all—of the required characteristics of a safeguards detector. This is currently driving the advances in technology.”
Nobel prizes are great, but what about stopping Iran from getting a nuclear weapon? What about the higher-than-normal background radiation Kay mentioned?
Antineutrinos, says Huber, “are sensitive only to material in a running reactor,” so while there is some concern of background noise, that noise can easily be accounted for, allowing researchers to safely “determine the plutonium content as well the enrichment level of uranium in the reactor core.” An antineutrino detection device, about the size of a trailer, might be vulnerable to computer hacking, like any device with microchips, but such a device would be incredibly difficult to spoof with fake radiation.
Antineutrino detection has never been a part of any weapons monitoring regime. Huber, working with researchers at Los Alamos National Laboratory, hopes to change that. They plan to deploy a prototype of a novel detector by the end of the year at a commercial reactor. With enough funding, Huber says that a fully calibrated detector could be ready to go Iran in two years (but one with a full safeguards system likely would not be available in fewer than five.)
Keep in mind, it only works when it’s deployed next to a known site. It won’t detect illicit nuclear activity across all of Iran. But if you can find an area that might be used for plutonium production, technically, you can verify with an atomic certainty how it is or isn’t being used.
NEXT STORY: The War On Terrorists’ Tweets